Research Trinity
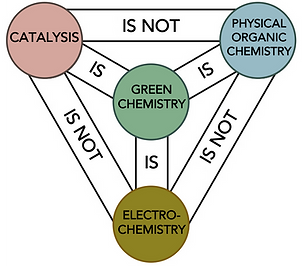

We develop robust and useful synthetic organic methods, and use physical organic chemistry tools to interrogate their reaction mechanisms.
Why?
The low-energy—do-nothing—pathway forward for humanity will inevitably be accompanied by a plethora of highly challenging scenarios. Thankfully, all around the world, efforts are being made to scale the high-energy barrier leading toward the ultimate utopia of global sustainability. However, the energy landscape is complex and multifaceted, and solutions are required for each of the innumerable systems that currently consume an excess of resources.
​
The pharmaceutical and agrochemical industries produce essential, high-value products. Unfortunately, the associated multi-step syntheses, frequent use of stoichiometric reagents and large solvent volumes result in high E-factors (mass of waste/mass of product). Thus, to ensure a sustained output of these important medicines and agrochemicals at reasonable costs, there is a demand to continually explore the efficiency of organic synthesis. The development of stream-lined and robust methodologies to prepare useful building-blocks and complex molecules from sustainable and inexpensive materials is highly important, and forms a central theme of research in the Lennox Lab.
We are specifically very interested in organofluorine compound, which are widely used in many important applications, including pharmaceuticals, agriculture, batteries and liquid crystals. For example, fluorine is used to modulate the function of pharmaceuticals as it can help to tune absorption, distribution, metabolism, and excretion (ADME) features of a drug. It can also productively influence conformation, pKa, intrinsic potency, membrane permeability, and metabolic stability. As organofluorine compounds are rare in nature, a lot of research effort is focussed on the regio and stereoselective incorporation of fluorine into complex organic molecules, which forms a nother central theme of research in the Lennox Lab.
How?
Our approach to achieving these goals involve the application of the latest state-of-the-art tools in reaction design, development and understanding.
We draw upon the fields of catalysis, organic electrochemistry and physical organic chemistry to aid reaction development. Catalysis provides a means of achieving a lower energy pathway, electrochemistry is an inherently sustainable and selective way to conduct redox reactions, and physical organic chemistry provides a fundamental understanding. As part of this, we apply computational calculations, machine learning algorithms, high throughput experimentation and reaction monitoring/kinetics techniques to enhance mechanistic understanding and reaction design.
​
We are convinced that the contribution of practical organic reactions and fluorination techniques, technological tools and mechanistic understanding will help transition the chemical industries toward a sustainable future.
​
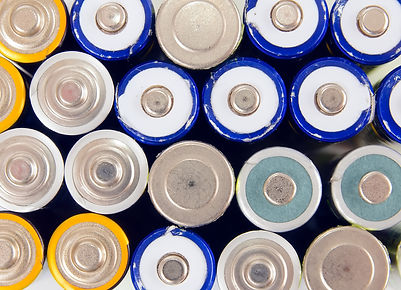
Hypervalent Iodine Mediated Alkene Fluorination
​
Hypervalent iodine (HVI) reagents are cost-effective, non-toxic, and environmentally benign reagents that are capable of mediating a broad spectrum of versatile reactivity. They can behave as oxidants and electrophilic reagents, but it is their ability to coordinate alkenes η² and activate them toward nucleophilic attack that has attracted interest from the Lennox Lab.
​

We have exploited this mode of alkene activation towards fluorination reactions. We have generated the HVI reagents using electrochemical oxidation, as it has proved to be an efficient and more sustainable way to generate these reagents.
​
Example publications:
​
Angew. Chem. Int. Ed., 2024, e202404666
Nat. Chem., 2024, 16,1647–1655
Hypervalent Iodine Mediated Alkyne Fluorination
​
Alkynes are more stable and less reactive than alkenes, and their corresponding functionalisation is therefore more challenging. We have been interested in the selective fluorination of alkynes for the past several years because the fluorinated alkene products are highly interesting and in general otherwise difficult to make. Monofluoroalkenes are bioisosteres for amides, which are the most widely used functional group in medicinal chemistry, hence, more ways to make different fluorinated alkenes is an important research goal.
We have approached this challenge with the use of hypervalent iodine reagents. We have developed a stereoselective method to prepare a key linchpin intermediate, called a Z-fluorovinyl iodonium salt (Z-FVI) that can be functionalised in several different ways to prepare different fluorinated alkenes.
​


Electrochemical Reduction
​
We are interested in the functionalisation of CF₃ groups. Conventionally, CF₃ groups are considered to be inert and unreactive. However, upon their single electron reduction, a defluorination event occurs to reveal a highly reactive intermediate that can be further functionalised. This strategy, not only opens the door towards the late stage functionalisation of complex molecules, but also is important for the remediation of perfluoroalkyl substances (PFAS) that do not readily biodegrade and therefore they bioaccumulate.
We are applying the use of electrochemical reduction to this challenge, because it is a sustainable way to apply the strongly reducing potentials that are required for this process to be successful.
We have also been advancing chemistry to prepare biaryl compounds using electrochemical reduction. This reaction highlights a promising alternative to traditional methods, potentially leading to safer and more cost-effective synthesis of biaryl compounds, which are relevant in the pharmaceutical industry.
​

Example publications:
​
JACS Au, 2024, 4, 6, 2220–2227
Angew. Chem. Int. Ed., 2023, e202218195
​
Electrochemical Oxidation
​
Our investigations in this area are motivated by the goal to functionalise C(sp³)–H bonds, which is an important strategy for the sustainable construction of complex molecules. We have been exploiting the use of electrochemical oxidation to generate arene radical cations can trap nucleophiles in the benzylic position. We have explored several different nucleophiles in this strategy, including carboxylic acids or nitriles. Fluoride has also been used as a nucleophile to form benzylic fluorides, and in this case the use of pulsed electrolysis waveforms was developed to enhance the yield and efficiency of the reaction. We found that pulsing the electrolysis improved mass transport, which limits over-oxidation.
​

3D printing reactors and electrodes
​
We have been advancing the development of 3D printed electrochemical reactors as a general strategy to improve the cost and reproducibility of electrochemical reactions. If reactors are 3D printed, they can be designed to perfectly suit the needs of the specific transformation. Then, the .stl files can be readily shared alongside the reporting of the reaction for others to be able to print and use themselves.
We have developed reactors for use with corrosive HF solutions, as well as to combine with the ElectraSyn, called the OpenESyn. We have also developed a user-friendly, open-source software tool called ERCAD, which aids the computational design process to enable non experts to readily produce their reactor designs for 3D printing.
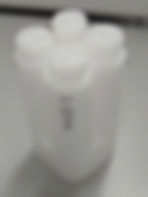


Example publications:
​
ChemElectroChem, 2021, 2070-2074
ChemElectroChem, 2024, e202400454
ACS Cent. Sci., 2024, 10, 11, 2028–2035
​
Funding is gratefully received from:




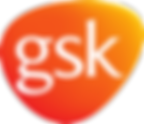

